Maybe its not all about practice. Since the youth sports world fell in love with the romantic notion that 10,000 hours of structured practice is the magic ingredient to world-class mastery in just about any field, especially sports, we've forgotten or ignored that our genetic endowment may still have something to do with the outcome. Just watch this video of a young Lionel Messi, who was probably still working towards his 10,000 hour total at the time. He clearly has something else, something that was already there at age 5 and something that the other kids didn't have.
David Epstein, senior writer at Sports Illustrated, has been on a search for that extra something. In his new book, The Sports Gene, Epstein launched himself directly into the nature vs. nurture, genes vs. practice and natural vs. self-made debates about athletic greatness.
I recently had a chance to chat with David about his book and found out that there is a complex, misunderstood mixture of variables in the magic formula:
David, congratulations on your new book! One of my all-time favorite SI articles of yours is the 2010 piece “Sports
Genes”. At the time, you opened many eyes on the influence of genetics on athletic
performance. Is it safe to say
that the science and our understanding of it has come a long way in the last
three years?
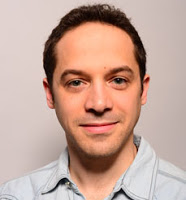 |
David Epstein |
David Epstein: I appreciate that! I think
it safe to say that the science has come a very long way in the last three
years. At the same time, the studies of genes related to sports performance is
still hampered by certain problems. A decade ago, scientists hoped that
genetics might be simple; that single traits, like, say, height, might be
attributable to a single gene or a small number of genes. But now it’s clear
that most traits—and certainly those as complex as athleticism—can involve
large numbers of genes, each with a small effect. That can make things
particularly tricky for studying elite athletes, because there aren’t very many
elite athletes in the world, so studies are often too small to detect the
effects of relevant genes.
Still, using certain innovative methods, like those
described in chapter five of my book, scientists are pinpointing some of the
genetic influences on an individual’s ability to adapt to a training regimen.
And that now looks to be a key component of “talent,” not simply some skill
that manifests prior to training, but the very biological setup that makes one
athlete better at adapting to a particular training plan. In recent years, both
with respect to endurance and strength training, the science has increasingly
shown that genes mediate the ability to “respond” to training, and it appears
that work will continue to be bolstered. People often say “I’m not very
talented in this or that area,” but the genetic work is increasingly showing
that we can’t necessarily know if we have talent before we try training.
In the book, you tell the story of Dan McLaughlin, an
amateur golfer, who has put his life on hold while he accumulates the infamous
10,000 hours of deliberate practice towards his goal of playing on the PGA
Tour. You document how genetics
can offer exclusive physical advantages for sprinters, swimmers or even
baseball batters. However, in
sports like golf, dominated more by mental skill than brute physical abilities,
does genetics still play a role or is it all about practice?
DE: That’s a great question. For
starters, there is less scientific evidence regarding genes that influence
skill in very technical sports, like golf, but that is partly because those
skills are difficult to study. We have enough trouble finding genes for simple
traits, like height, and physiologists don’t even understand everything that
makes a great golfer, much less the genes that undergird the particular
physiological traits. As Sir Roger Bannister once said: “The human body is
centuries in advance of the physiologist, and can perform an integration of
heart, lungs, and muscles which is too complex for the scientist to analyze.”
No where is this complexity more difficult for scientists to link to specific
traits than in sports based on specialized skills. So one reason there’s more
known about genes—or innate physiological traits—that influence the more raw
athletic skills is simply because scientists more often choose to study
athletes engaged in more “raw” sports. The idea is it will be easier to find
the biological influences.
That said, there are mounds of studies that show
that when individuals practice motor skills, differences in the rate of
progress become apparent in all but extremely simple skills. In some studies,
the more complex the skill, the greater the differences between individuals
will become as they practice. In other words, there are differences in
“trainability.” Which genes are at play here is largely a mystery, but that doesn’t
mean they don’t exist. Remember, we don’t know many of the genes that influence
height, and yet from studies of families and large populations, we know quite
well that differences in the heights of adults in any given population are
generally at least 80% inherited.
To use an example relevant to some of the
writing in my book, left-handed people are highly overrepresented among chess
masters. We don’t know what the “left handed genes” are, but we know there is a
genetic component. Men are about twice as likely to be left handed as women,
for example. So it would seem as if certain genes for left-handedness, which of
course means brains that influence motor control in the brain, interact with
the learning of a skill like chess. As a related aside, Belgian scientist
Debbie Van Beisen has shown that competitive table tennis players with mental
handicaps fail to learn the anticipatory cues required to return shots as
quickly as similarly experienced table tennis players who do not have mental
handicaps.
Additionally (and I actually had
to trim much of this from the book) there is some interesting work implicating
specific genes in motor skill learning. Here’s a snippet I had to cut from the
book, as my first draft was WAY over printable length:
“The level of BDNF is
elevated in the brain’s motor cortex when people learn a motor skill, and BDNF
is one of the neural signals that coordinates the reorganization of the brain
when skills are learned. And a 2006 study found that, when people practiced
motor skills with their right hand—like putting small pegs in holes as quickly
as possible—the area of the activated brain representing the right hand, the
neural “motor map,” increased in size with practice only in those people who
did not have a met version of the BDNF gene. All of the subjects started with
similar sized motor maps, but only the non-met carriers experienced a change
with practice.
And in 2010 a group of
scientists led by neurologist Steven C. Cramer set out explicitly to test
whether the BDNF gene impacts the kind of memory involved in motor skill
learning, and their findings suggest that it does. In that study, people drove
a car along a digital track 15 separate times in one day. All of the drivers
improved as they learned the course, but the met carriers did not improve as
much. And when all the drivers were asked back four days hence and made to
drive the course once more, the met carriers made more mistakes. When
scientists used fMRI to look at the drivers’ brains as they practiced simple
motor skills, they found different patterns of activation in the people who had
a met version of the BDNF gene.”
Recently, Atlas Sports Genetics has caused a stir in youth
sports by offering parents a test for their kids to look for a certain
variation of the ACTN3 gene, otherwise known as the “speed gene.” You mention that this test is only
useful to know if your youngster is the next Usain Bolt or Carmelita Jeter,
something parents probably already know.
What’s next on the horizon for genetic testing for young athletes? Are there genes or combinations of
genes for traits like reaction time, balance or coordination?
DE: First, just to clarify, the
ACTN3 gene is only really useful for telling you that your youngster will not be the next Bolt—if they don’t have
the so-called “right” version for sprinting. But it doesn’t even do a very
specific job of that, since most people have the “right” version. And, let’s
face it, you can take your kid to the playground and have him race the other
kids and you’ll get a better idea of his chances of becoming the next Bolt than
you would with a genetic test.
As far as the next frontier of genetic testing
for young athletes, I think it will undoubtedly be “injury genes,” before
performance genes, and we’re already actually starting to see a bit of that. I
spent some time with Brandon Colby, an L.A.-based physician who treats retired
NFL players, and—at the behest of parents—he already tests some teenagers for
their version of the ApoE gene. As I write in the book, one version of this
gene makes an individual more susceptible to brain damage from concussions or
the kind of hits to the head to occur on every football play. There are other
gene variants that put some athletes at risk of dropping dead on the field, and
others that appear to increase the risk of an injury like a ruptured Achilles
tendon or torn ACL.
As I discuss in the book, some of these genes are actually
now being used for practical purposes, and I think that we’ll see that
increase. As for reaction time, I don’t think we’ll see much there, given that,
as I explain in the first chapter, the simple reaction times—the time it takes
one to hit a button in response to a light—of elite athletes are no different
than those of teachers, lawyers, or college kids. The skills that allow hitters
to intercept 100 mph fastballs are learned perceptual skills, not innate
reaction abilities. And even if simple reaction time was important, it would be
way easier to measure directly—by giving someone a reaction time test—than
indirectly by looking at genes.
Here at Sports Are 80 Percent Mental, we talk a lot about the
brain’s role in playing sports.
From vision to perception to decision making to emotions, the brain
plays a critical role in sports success.
What have we learned about neurogenetics that can influence an athlete’s
performance from a cognitive perspective?
DE: One of the most surprising
things I learned in my reporting was that scientists know quite well that not
only does the dopamine system in the brain—which is involved in the sense of
pleasure and reward—respond to physical activity, but it can also drive
physical activity.
One of the scientists I quote in the book suggests that this
may be why very active children who take Ritalin, which alters dopamine levels,
suddenly have less drive to move around. That’s precisely what he sees when he
gives Ritalin to the rodents he breeds for high voluntary running, anyway. And
it appears that different versions of genes involved in the dopamine system influence
the drive to be active. (Interestingly, native populations that are nomadic and
that migrate long distances tend to have a higher prevalence of a particular
dopamine receptor gene; the same one that predisposes people to ADHD. I discuss
in the book the possible link.)
One of my takeaways from the research I did for
the book was that some traits we think are innate, like the bullet-fast
reactions of a Major League hitter, are not, and others that we often portray
as entirely voluntary—like the compulsive drive to train—can have important
genetic components. Additionally, the section of the book that deals with pain
in sports, and discusses the genetics of pain, gets into the fact that the
circuitry of pain is shared with circuitry of emotion. (Morphine, after all,
doesn’t so much dull pain as make one less upset about it.) And the first genes
that are emerging that might allow athletes to deal calmly with pain on the
field—like, perhaps, the COMT “warrior/worrier” gene—are genes involved in the
metabolism of neurotransmitters in the brain. And, of course, as I mentioned in
my longwinded answer to the second question, there are genes that appear to
influence motor learning.
David, you were a competitive runner in your college days at
Columbia and I understand you still run quite a bit. Has the research for this book given you any insight or tips
that you or other weekend athletes can use?
DE: Indeed I was. I was an
800-meter runner. I still love running, but I’d call what I do now “jogging”!
But working on this book gave me certain broad insights that I apply to my own
training. In 2007, the prestigious peer-reviewed journal Science listed “human genetic variation” as the breakthrough of the
year; the revelation of how truly different we are from one another. And, as
J.M. Tanner, the eminent growth expert (and world class hurdler) once put it:
“Everyone has a different genotype. Therefore, for optimal development…everyone
should have a different environment.” No two people respond to a Tylenol the
same way because of their distinct biology, and no two people respond to the
medicine of exercise the same way either.
When I was in college, I had better
endurance—at all distances—on a training plan of 35 miles per week that
included carefully selected intervals, than I had previously on 85 miles per
week of cookie-cutter distance training. If you aren’t taking a scientific
approach to your training—and this doesn’t mean cutting edge science, but just
paying attention to what you best respond to—then you aren’t getting everything
out of yourself. To use track, because it’s just an easy example, in every
training group from high school to the pros, you have groups of runners doing
identical workouts, and yet never crossing the line at the same time in a race.
Genetic science is showing us that the most important kind of “talent” isn’t
some physical trait that preexists training, but rather that ability to
physically adapt to training. And studies I describe in the book make it quite
clear that particular genes mediate an individual’s ability to benefit from
training such that two people can have drastically different results from the
same training plan.
So if you feel like, for some reason, you aren’t getting
results on par with your training partner, you might be right. And the problem
might be you, in the very deepest
sense. So don’t be afraid to try something different. Several of the athletes I
write about in the book weren’t afraid to jump into entirely new activities or
training plans, and some came out world champions.
Thank you, David and good luck with the book!